Lead (Pb) Ore
Lead ore is a naturally occurring mineral deposit that contains lead in varying concentrations. It is typically extracted from the Earth’s crust through mining and is an important source of lead, which is a soft, heavy, and bluish-gray metal. Lead ore is typically found in association with other minerals and rocks in various geological formations and is widely distributed around the world.

Lead ore is primarily composed of lead sulfide (PbS), which is the most common mineral form of lead. Other minerals that may be present in lead ore deposits include cerussite (lead carbonate, PbCO3), anglesite (lead sulfate, PbSO4), galenite (lead sulfide, PbS), and other lead-bearing minerals. The concentration of lead in lead ore deposits can vary widely, ranging from a few percent to several tens of percent, depending on the deposit’s geology and mineralogy.
Lead ore has been used by humans for thousands of years for various purposes, including as a source of metal for manufacturing, construction, and other industrial applications. However, due to its toxicity, lead has also been associated with environmental and health concerns, and regulations regarding its mining, processing, and usage have been implemented to protect human health and the environment.
Contents
Lead Ore Properties
Lead ore, also known as galena, is a naturally occurring mineral composed of lead sulfide (PbS). It is the primary ore of lead and is commonly found in various geological formations around the world. Here are some key properties of lead ore:
Chemical Formula: PbS Hardness: 2.5-2.75 on the Mohs scale Density: 7.2-7.6 g/cm³ Color: Typically gray to silver-gray, may have a metallic luster Crystal System: Cubic Cleavage: Perfect cubic cleavage in three directions Fracture: Subconchoidal to uneven Streak: Lead-gray Luster: Metallic Transparency: Opaque Specific Gravity: 7.4-7.6 Magnetic Properties: Non-magnetic Other Properties: Lead ore is soft, heavy, and has a relatively low melting point of around 327°C.
Lead ore is an important source of lead, which is a soft, malleable, and dense metal with many industrial applications. Lead has been used by humans for thousands of years in various applications, including batteries, ammunition, construction, and as a stabilizer in plastics, among others. However, due to its toxic properties, lead exposure can have harmful effects on human health and the environment, and appropriate safety measures must be taken during mining, processing, and use of lead ore and lead-containing products.
It’s important to note that properties of lead ore can vary depending on the specific geological formation and location from which it is extracted. Different impurities, mineral associations, and geological conditions can impact the properties of lead ore, and detailed characterization through geological and mineralogical analysis is necessary for accurate understanding of lead ore properties in a specific deposit or location.
Lead Ore Minerals
Lead ores typically contain lead minerals, which are naturally occurring compounds that contain lead as a major component. Some of the common lead minerals found in lead ore deposits include:
- Galena (PbS): Galena is the most common and important lead mineral, and it is typically the primary ore mineral in lead deposits. Galena is a lead sulfide mineral that crystallizes in the cubic crystal system and has a bright metallic luster. It is often found in well-formed crystals and can be gray, silver-gray, or black in color.
- Cerussite (PbCO3): Cerussite is a lead carbonate mineral that forms in the oxidized zone of lead ore deposits. It is typically found as prismatic crystals or granular masses and can be colorless, white, or gray in color. Cerussite is less common than galena but can be an important secondary lead mineral in some deposits.
- Anglesite (PbSO4): Anglesite is a lead sulfate mineral that forms in the oxidized zone of lead ore deposits. It is typically found as prismatic crystals or fibrous masses and can be colorless, white, or gray in color. Anglesite is also less common than galena but can be an important secondary lead mineral in some deposits.
- Pyromorphite (Pb5(PO4)3Cl): Pyromorphite is a lead phosphate mineral that forms in the oxidized zone of lead ore deposits. It is typically found as prismatic crystals or botryoidal masses and can be green, brown, or yellow in color. Pyromorphite is less common than galena, cerussite, and anglesite, but it can be present in some lead ore deposits as a secondary mineral.
- Other Lead Minerals: Other less common lead minerals found in lead ore deposits include mimetite (Pb5(AsO4)3Cl), vanadinite (Pb5(VO4)3Cl), and wulfenite (Pb(MoO4)). These minerals can occur as secondary lead minerals in oxidized zones of lead deposits and can exhibit a range of colors, including yellow, brown, green, or red.
It’s important to note that the mineralogy of lead ores can vary depending on the specific deposit and its geological conditions. The above-listed minerals are some of the common lead minerals found in lead ore deposits, but there may be other minerals associated with lead ores as well. Detailed mineralogical studies and analysis are typically conducted during exploration and evaluation of lead deposits to accurately identify and quantify the lead minerals present.
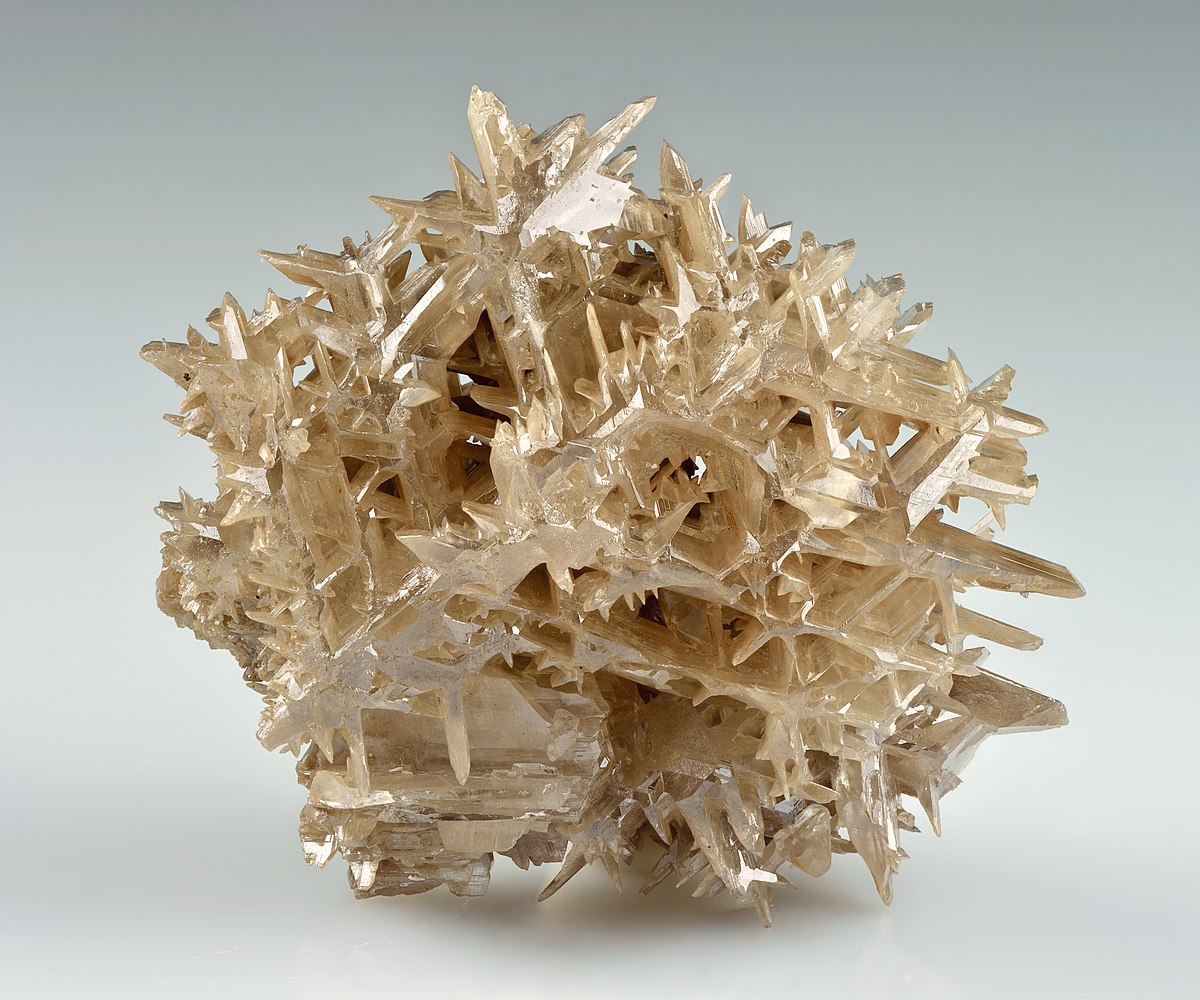
Geology and Formation of Lead Ore Deposits
Lead ore deposits are typically formed through various geological processes involving the interaction of mineral-rich fluids with host rocks. The geology and formation of lead ore deposits can vary depending on the type of deposit and its specific geological setting. However, some general processes and geological features associated with the formation of lead ore deposits include:
- Hydrothermal processes: Many lead ore deposits are formed through hydrothermal processes, where hot, mineral-rich fluids are circulated through fractures and faults in the Earth’s crust. These fluids can dissolve lead and other minerals from surrounding rocks and deposit them in open spaces, such as veins or pockets, as they cool and precipitate. Hydrothermal lead ore deposits can occur in a variety of rock types, including sedimentary, igneous, and metamorphic rocks.
- Sedimentary processes: Lead ore deposits can also form through sedimentary processes, where lead minerals are deposited as sediments in ancient marine or lacustrine environments. Over time, these sediments can undergo compaction, cementation, and diagenesis, leading to the formation of lead ore deposits. Sedimentary lead ore deposits can be found in sedimentary rocks, such as limestone, dolomite, and shale, and are often associated with other minerals and organic matter.
- Replacement processes: In some cases, lead ore deposits can form through replacement processes, where lead minerals replace existing minerals in pre-existing rocks. This can occur through chemical reactions between lead-rich fluids and host rocks, resulting in the replacement of original minerals with lead minerals. Replacement lead ore deposits can be found in a variety of rock types, including carbonate rocks, sulfide-rich rocks, and silicate rocks.
- Weathering and oxidation processes: Weathering and oxidation processes can also contribute to the formation of lead ore deposits. In oxidized zones near the Earth’s surface, lead minerals can be altered through weathering and oxidation, resulting in the formation of secondary lead minerals, such as cerussite and anglesite. These secondary lead minerals can accumulate in the weathered zone, forming lead ore deposits.
- Tectonic and structural controls: Tectonic and structural features, such as faults, folds, and fractures, can play a significant role in the formation and localization of lead ore deposits. These features can create pathways for mineral-rich fluids to circulate and deposit lead minerals, resulting in the formation of lead ore deposits along or near these structures.
It’s important to note that the formation of lead ore deposits is a complex process influenced by various geological factors, including rock type, mineralization styles, fluid chemistry, temperature, pressure, and time. The geology and formation of lead ore deposits can vary significantly from deposit to deposit, and detailed geological studies and exploration methods are typically used to understand the specific geology and formation processes of lead ore deposits in a given area.
Exploration and Evaluation of Lead Ore Deposits
The exploration and evaluation of lead ore deposits typically involves a multi-stage process that includes various geological, geochemical, and geophysical techniques to identify prospective areas and assess the potential of lead mineralization. Here are some general steps that may be involved in the exploration and evaluation of lead ore deposits:
- Desk-based studies: The first step in exploring for lead ore deposits is typically a review of existing geological, geochemical, and geophysical data, as well as historical mining records, to identify prospective areas. This can involve compiling and analyzing data from maps, reports, and databases, as well as conducting literature reviews and consulting with experts in the field.
- Geological mapping and sampling: Field-based geological mapping and sampling are important steps in the exploration process. This involves conducting detailed geological mapping of the target area to identify rock types, structures, and alteration patterns that may be indicative of lead mineralization. Rock samples may be collected for laboratory analysis to determine their geochemical composition, mineralogy, and potential for lead mineralization.
- Geophysical surveys: Geophysical surveys are commonly used in lead ore exploration to identify subsurface features that may be associated with lead mineralization. Techniques such as magnetic, gravity, and electromagnetic surveys can help identify areas with anomalous signatures that may indicate the presence of lead ore deposits.
- Geochemical surveys: Geochemical surveys involve collecting and analyzing soil, rock, and water samples for their geochemical composition, including lead and other associated elements. Geochemical surveys can help identify areas with elevated levels of lead or other pathfinder elements that may indicate the presence of lead mineralization.
- Diamond drilling: Diamond drilling is a common method used to obtain core samples from the subsurface for detailed geological, geochemical, and mineralogical analysis. Diamond drilling can provide valuable information about the composition, structure, and characteristics of the rocks and minerals in the target area, helping to assess the potential for lead ore deposits.
- Mineralogical studies: Detailed mineralogical studies of rock samples, including thin section analysis, X-ray diffraction (XRD), and scanning electron microscopy (SEM), can provide important information about the types, distribution, and characteristics of lead minerals in the target area.
- Economic evaluation: Once sufficient data has been gathered, an economic evaluation can be conducted to assess the potential viability of a lead ore deposit. This may involve estimating the size, grade, and tonnage of the lead mineralization, as well as evaluating factors such as infrastructure, logistics, metallurgical processing, and market conditions to determine the economic feasibility of developing the deposit.
It’s important to note that the exploration and evaluation process for lead ore deposits can be complex and may require the expertise of geologists, geochemists, geophysicists, and other specialists. The specific methods and techniques used can vary depending on the geological setting, size of the target area, available data, and exploration budget. Proper permitting, environmental considerations, and health and safety measures should also be followed throughout the exploration and evaluation process.
Mining and Processing of Lead Ores
Mining and processing of lead ores typically involves several stages, including extraction, beneficiation, and smelting. Here is a general overview of the mining and processing process for lead ores:
- Extraction: The first step in mining lead ores is to extract the ore from the earth. This can be done through various methods depending on the type and location of the deposit. Common methods include open-pit or underground mining, depending on the depth and accessibility of the deposit. Once the ore is extracted, it is typically transported to the surface for further processing.
- Beneficiation: After the ore is extracted, it is usually subjected to beneficiation, which involves crushing, grinding, and separating the ore from the waste rock and other impurities. This is done to increase the concentration of lead minerals in the ore, making it easier to extract and process further. Beneficiation may also include froth flotation, magnetic separation, or other methods to separate the lead minerals from other minerals.
- Smelting: Smelting is the process of extracting lead from the concentrated ore. The concentrated lead ore is usually roasted in a furnace to remove impurities, and then mixed with coke (a form of carbon) and limestone (a fluxing agent) in a smelting furnace. The mixture is heated to high temperatures, causing the lead minerals to melt and separate from the impurities. The molten lead is then tapped from the bottom of the furnace and cast into molds to form lead bullion.
- Refining: Lead bullion obtained from the smelting process may contain impurities such as copper, silver, and other metals. It is further refined through processes such as electrorefining or cupellation to remove these impurities and produce high-purity lead.
- Environmental considerations: Mining and processing of lead ores can have environmental impacts, including air and water pollution, habitat destruction, and soil contamination. Proper environmental management practices, including waste disposal, water management, air emissions control, and site reclamation, are important considerations in the mining and processing of lead ores to minimize the impact on the environment and surrounding communities.
- Occupational health and safety: Lead mining and processing can also pose health and safety risks to workers, including exposure to lead dust, fumes, and other hazardous substances. Proper safety measures, including personal protective equipment (PPE), ventilation, and training, should be implemented to protect the health and safety of workers.
- Regulatory compliance: Mining and processing of lead ores are subject to various regulatory requirements and permits, including environmental permits, mining licenses, and occupational health and safety regulations. Compliance with these regulations is important to ensure responsible and sustainable mining and processing practices.
It’s important to note that the specific methods and processes used in mining and processing of lead ores can vary depending on the type of deposit, location, and technological advancements. Additionally, proper management of waste and byproducts, such as tailings and slag, should be followed to minimize environmental impacts and ensure responsible mining and processing practices.

Uses of Lead and Lead Products
Lead has been used by humans for thousands of years due to its versatile properties. Some common uses of lead and lead products include:
- Batteries: Lead-acid batteries are widely used in various applications, including automotive batteries for vehicles, backup power supplies, uninterruptible power supply (UPS) systems, and more. Lead’s high density, low melting point, and ability to be easily formed into different shapes make it ideal for battery production.
- Construction and Plumbing: Lead has been used in the construction and plumbing industry for many years due to its malleability, durability, and resistance to corrosion. Lead-based products such as lead sheets, lead pipes, and lead flashing are used in roofing, cladding, gutters, and other applications.
- Ammunition: Lead has been used in bullets and shot for ammunition due to its high density and ability to be easily formed into projectiles. However, the use of lead in ammunition is increasingly being regulated and phased out in some jurisdictions due to environmental concerns related to lead contamination.
- Radiation Shielding: Lead is used as a shielding material in various applications involving radiation, such as in medical facilities, nuclear power plants, and industrial settings. Lead’s high density and ability to absorb and block radiation make it an effective shielding material.
- Electronics: Lead has been used in the production of electronics, particularly in soldering. However, the use of lead in electronics has been regulated in many countries due to concerns about environmental contamination and potential health risks, leading to the development of lead-free soldering technologies.
- Weight Balancing: Lead is used in weight balancing applications, such as in the production of weights for balance wheels in machinery and equipment, as well as in sports equipment like scuba diving belts and fishing sinkers.
- Pigments: Lead compounds, such as lead oxide and lead chromate, have been used historically in the production of pigments for paints, ceramics, and other applications. However, the use of lead-based pigments has declined in recent years due to environmental and health concerns, and alternative pigments are now commonly used.
- Other Applications: Lead has also been used in a variety of other applications, including as an additive in some types of glass, as a component in certain types of solders and alloys, and in the production of certain chemicals and materials.
It’s important to note that the use of lead and lead products is subject to regulatory requirements and restrictions in many countries due to concerns about environmental contamination and health risks associated with lead exposure. Proper handling, use, and disposal of lead and lead-containing products are crucial to prevent environmental pollution and protect human health.

Occurrence and Distribution of Lead Ores Worldwide
Lead ore deposits are found in various regions around the world, with significant occurrences in several countries. The occurrence and distribution of lead ores worldwide can vary depending on geological, mineralogical, and economic factors. Here is an overview of the occurrence and distribution of lead ores in different regions:
- Australia: Lead ore deposits are found in various regions of Australia, including the Broken Hill region in New South Wales, Mount Isa region in Queensland, and Western Australia. These deposits are typically associated with other base metal ores, such as zinc and silver, and occur in a variety of geological settings, including sedimentary, volcanic, and metamorphic rocks.
- United States: Lead ore deposits are found in several states in the United States, including Missouri, Idaho, Alaska, and Colorado. The Missouri lead belt, located in the southeastern part of the state, is one of the major lead-producing regions in the world, known for its extensive lead-zinc deposits hosted in sedimentary rocks.
- China: China is one of the largest producers of lead ores in the world, with significant deposits found in various provinces, including Yunnan, Henan, Hunan, and Inner Mongolia. These deposits are typically associated with other base metal ores and occur in diverse geological settings, including sedimentary, volcanic, and hydrothermal deposits.
- Peru: Peru is another major producer of lead ores, with deposits located in the central Andes mountain range. The Cerro de Pasco region in central Peru is known for its lead-zinc-silver deposits hosted in carbonate rocks.
- Canada: Lead ores are found in several regions of Canada, including the Bathurst Mining Camp in New Brunswick, the Sullivan Mine in British Columbia, and the Pine Point Mine in Northwest Territories. These deposits are typically associated with other base metal ores, such as zinc and copper, and occur in various geological settings, including sedimentary, volcanic, and metamorphic rocks.
- Other Countries: Lead ores are also found in other countries, such as Mexico, Russia, India, Kazakhstan, Sweden, Spain, Morocco, and many others, although the production levels may vary.
It’s important to note that the occurrence and distribution of lead ores can change over time due to exploration discoveries, economic factors, and environmental regulations. The information provided here is a general overview and may not be exhaustive or up-to-date. Further research and reference to reliable sources are recommended for a comprehensive understanding of the occurrence and distribution of lead ores worldwide.
Market Trends and Challenges in the Lead Ore Industry
The lead ore industry, like other mineral industries, is influenced by various market trends and faces challenges that impact its production, demand, and overall outlook. Some of the market trends and challenges in the lead ore industry include:
- Global Demand: The demand for lead ore is influenced by various factors, including economic growth, industrial production, and infrastructure development. Global demand for lead has been relatively stable in recent years, with increasing demand from emerging economies offsetting declining demand from developed countries.
- Environmental Regulations: The lead ore industry is subject to strict environmental regulations in many countries due to concerns about lead contamination and its impact on human health and the environment. These regulations may include restrictions on lead mining, processing, and emissions, as well as requirements for environmental monitoring, remediation, and rehabilitation.
- Health and Safety Concerns: The lead ore industry has long been associated with health and safety concerns, as lead exposure can pose significant risks to human health, especially to workers involved in mining, processing, and other operations. Ensuring proper occupational health and safety measures, including proper ventilation, personal protective equipment, and lead exposure monitoring, is crucial to protect workers’ health and safety.
- Recycling and Substitution: Lead is a recyclable material, and the increasing emphasis on sustainability and circular economy has led to growing interest in lead recycling. Recycling of lead-based products, such as lead-acid batteries, can help reduce the demand for primary lead ore. Additionally, there is ongoing research and development in finding substitutes for lead in various applications, which could impact the future demand for lead ore.
- Technological Advancements: Technological advancements, such as improvements in mining and processing techniques, can impact the production and cost-effectiveness of lead ore. Advancements in exploration methods, mining equipment, and processing technologies can improve the efficiency and sustainability of lead ore operations.
- Economic Factors: Economic factors, such as fluctuations in global commodity prices, exchange rates, and geopolitical tensions, can impact the lead ore market. Volatility in metal prices can influence the profitability of lead ore operations, as well as investment decisions and exploration activities.
- Social and Community Impacts: Lead ore mining and processing can have social and community impacts, including displacement of communities, land use conflicts, and potential impacts on local water resources and ecosystems. Ensuring responsible mining practices, community engagement, and sustainable resource management are important considerations for the lead ore industry.
- Supply Chain and Trade Dynamics: The lead ore industry is part of a global supply chain, with lead ores being traded and transported internationally. Trade dynamics, including tariffs, trade policies, and transportation costs, can impact the competitiveness and profitability of lead ore producers.
- Geopolitical Factors: Geopolitical factors, such as changes in trade policies, sanctions, and political stability in lead ore-producing countries, can impact the supply and demand dynamics of the lead ore market. Geopolitical events can result in disruptions to the supply chain and affect the availability and price of lead ore in global markets.
Navigating these market trends and challenges requires careful planning, technological innovation, sustainable practices, and adherence to regulatory requirements. The lead ore industry needs to adapt to changing market dynamics, address environmental and health concerns, and ensure responsible mining and processing practices to sustainably meet global demand for lead products.