Bastnäsite is a group of rare earth fluorocarbonate minerals that includes bastnäsite-(Ce), bastnäsite-(La), and bastnäsite-(Y), with each type named after the dominant rare earth element it contains (cerium, lanthanum, and yttrium, respectively). These minerals are typically brown, reddish-brown, or yellowish in color and have a glassy to resinous luster. They are primarily found in carbonate-rich hydrothermal deposits, often associated with other rare earth minerals like monazite and xenotime.
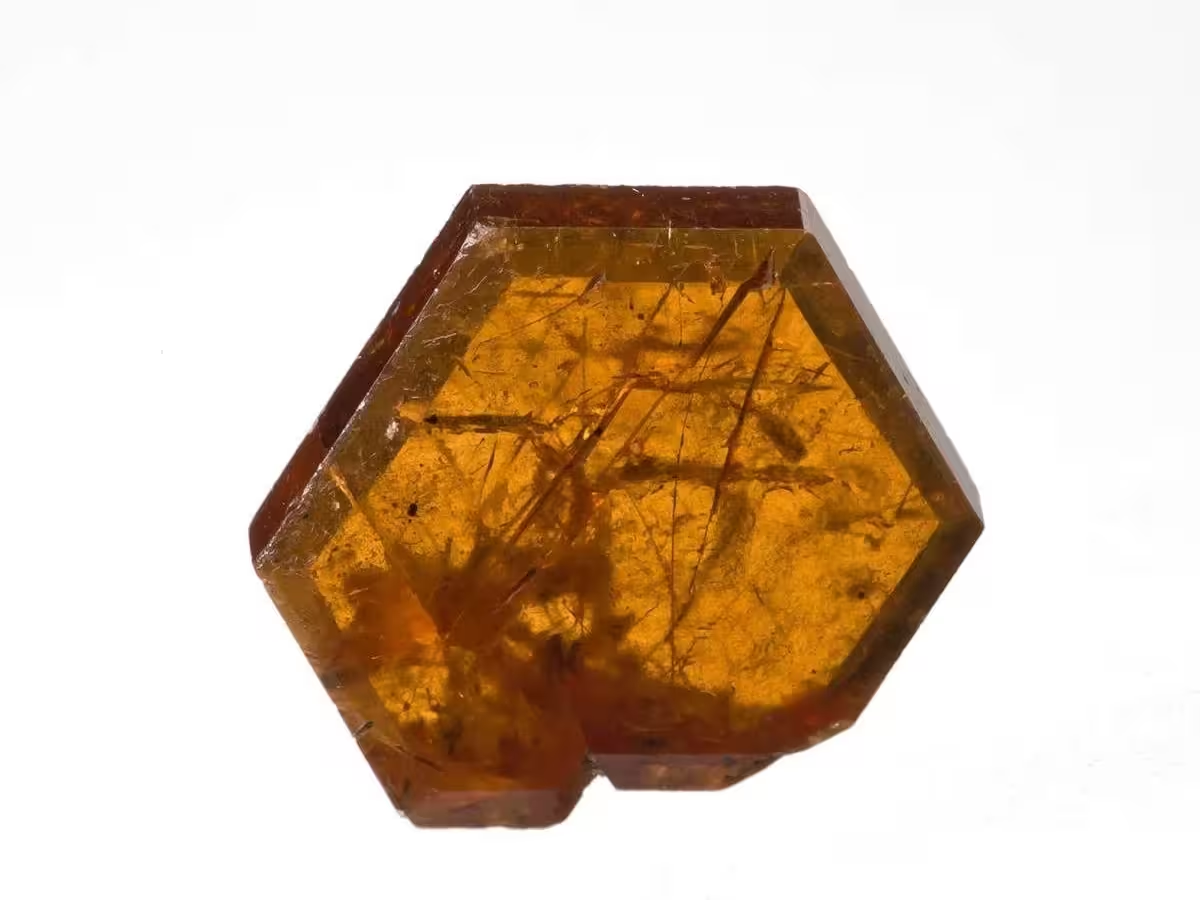
History and Discovery
Bastnäsite was first discovered in 1838 in Bastnäs, Sweden, which is where it derives its name. It was identified in the tailings of the Bastnäs mines, which were primarily mined for copper and cobalt. The unique properties of bastnäsite, such as its high content of cerium and other rare earth elements, led to increased interest in the mineral. Since its discovery, bastnäsite deposits have been found in other locations worldwide, including China, the United States, and Madagascar.
Importance in the Context of Rare Earth Elements
Bastnäsite is a crucial source of rare earth elements (REEs), particularly light rare earth elements (LREEs) such as cerium, lanthanum, and neodymium. These elements are essential for various high-tech applications, including the production of permanent magnets, catalytic converters, and phosphors for lighting and displays. Bastnäsite deposits are significant because they often contain high concentrations of REEs, making them economically viable for mining and processing. The mineral has become increasingly important due to the growing demand for REEs in renewable energy technologies, electronics, and other advanced industries. As a result, bastnäsite plays a key role in global supply chains for critical materials.
Contents
Chemical Composition and Structure of Bastnäsite
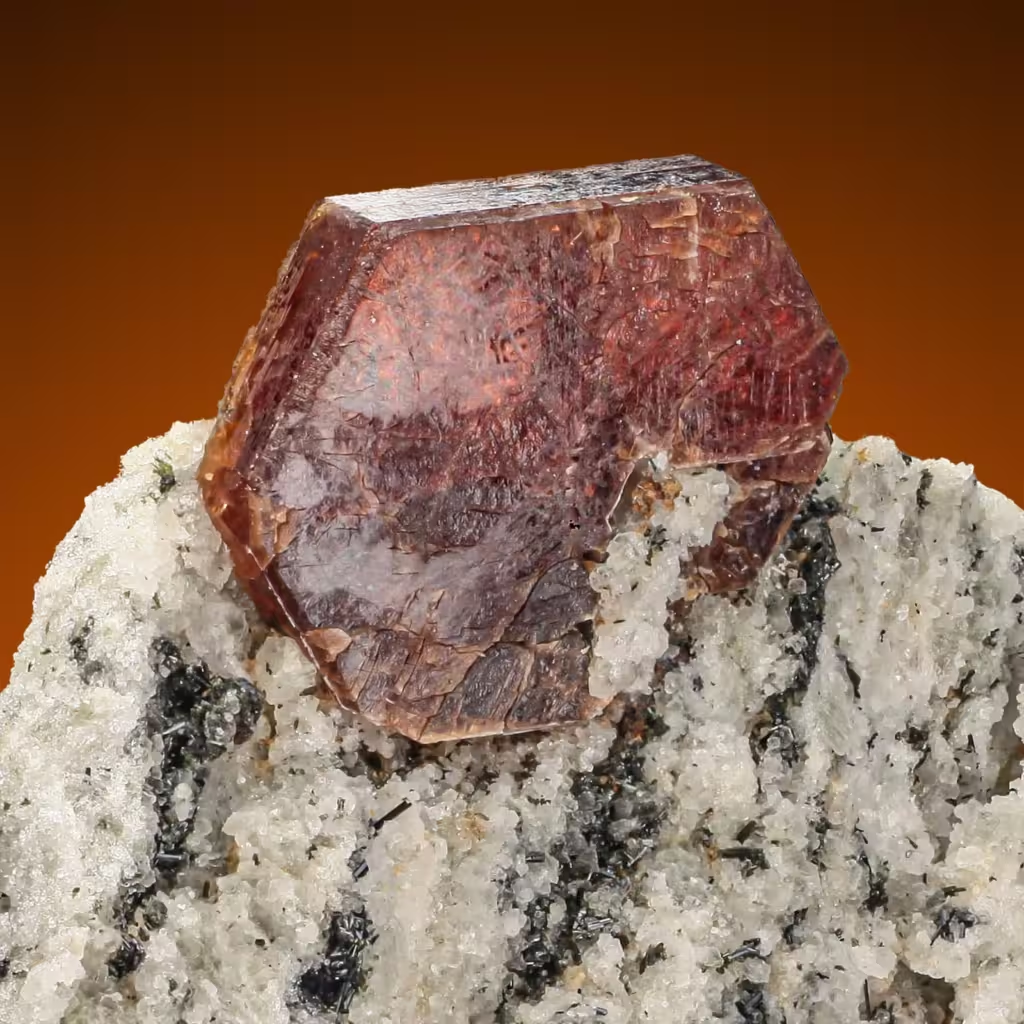
Chemical Composition
Bastnäsite is a fluorocarbonate mineral with the general chemical formula (REE)CO3F\text{(REE)}\text{CO}_3\text{F}(REE)CO3F, where “REE” represents rare earth elements, primarily cerium (Ce), lanthanum (La), and yttrium (Y). The specific composition can vary based on the dominant rare earth element, resulting in different varieties of the mineral:
- Bastnäsite-(Ce): CeCO3F\text{CeCO}_3\text{F}CeCO3F
- Bastnäsite-(La): LaCO3F\text{LaCO}_3\text{F}LaCO3F
- Bastnäsite-(Y): YCO3F\text{YCO}_3\text{F}YCO3F
In addition to these primary elements, bastnäsite may also contain trace amounts of other rare earth elements such as neodymium (Nd), praseodymium (Pr), and samarium (Sm). The presence of fluorine (F) in its structure distinguishes it from other rare earth minerals like monazite and xenotime, which are phosphates.
Crystal Structure
Bastnäsite crystallizes in the hexagonal crystal system, specifically in the space group P63/mmcP6_3/mmcP63/mmc. Its structure consists of layers of rare earth cations (such as Ce, La, or Y) coordinated to oxygen atoms from the carbonate groups (CO32−\text{CO}_3^{2-}CO32−), with the carbonate planes alternating with layers containing fluorine atoms. The carbonate groups are trigonal planar, and each REE cation is surrounded by nine oxygen atoms forming a tricapped trigonal prismatic coordination geometry.
This layered structure allows for significant flexibility in the mineral’s composition, accommodating various rare earth elements in the crystal lattice. The presence of fluorine further stabilizes the structure, influencing the mineral’s physical properties, such as its relative softness (Mohs hardness of 4–4.5) and its cleavage properties.
Structural Implications
The unique structure of bastnäsite contributes to its importance as a source of rare earth elements. The presence of large cations like cerium and lanthanum in the lattice allows for relatively easy extraction of these elements during mining and processing. Additionally, the mineral’s stability in hydrothermal environments makes it an excellent indicator of geological processes associated with rare earth element concentrations, contributing to the understanding of REE ore formation.
Geological Occurrence of Bastnäsite
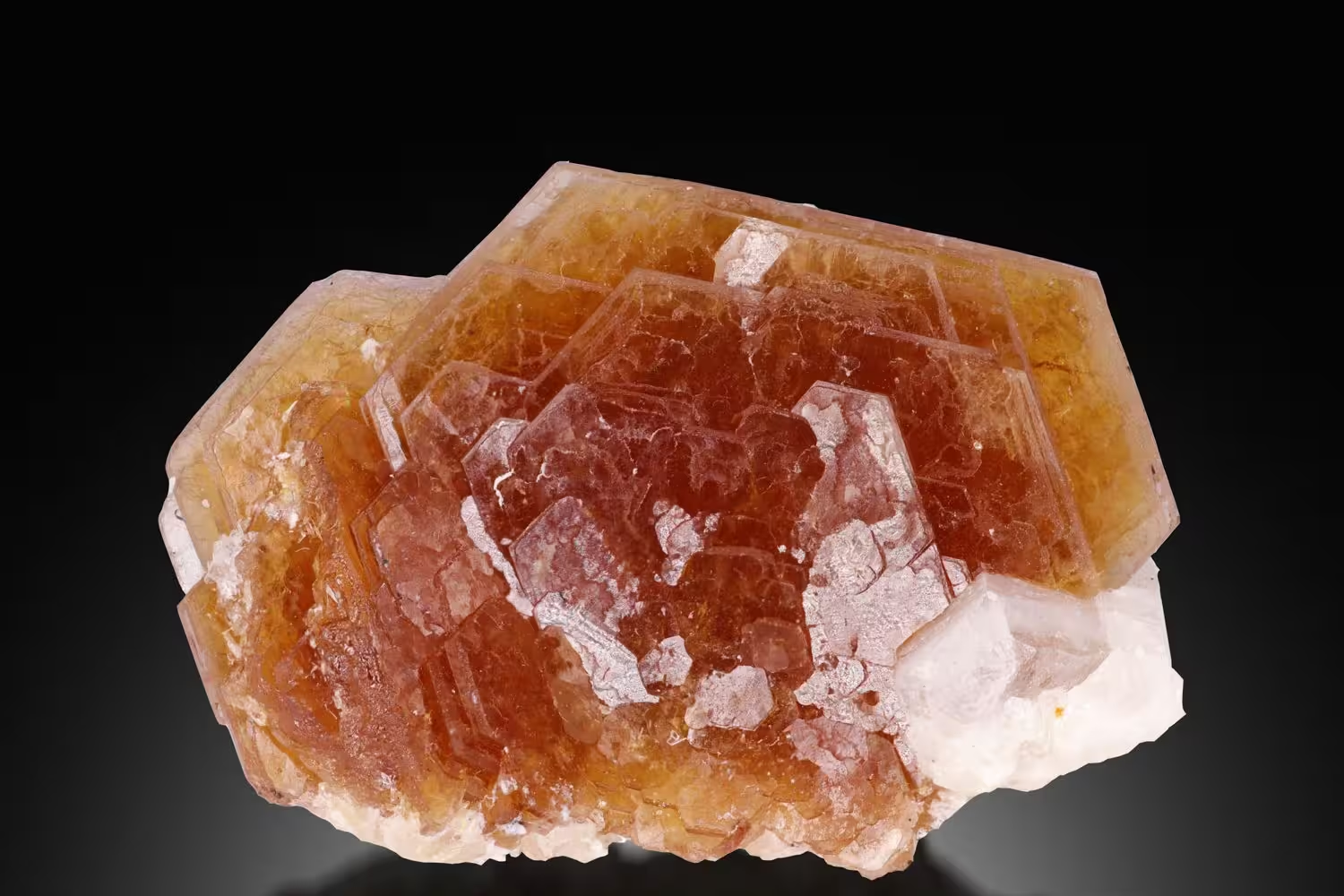
Types of Deposits
Bastnäsite is primarily found in two types of deposits: primary and secondary.
- Primary Deposits:
Primary bastnäsite deposits are associated with carbonatite and alkaline igneous complexes. These deposits form when rare earth elements are concentrated by magmatic processes, particularly in carbonate-rich magmas. As these magmas cool and crystallize, bastnäsite forms as a primary mineral, often along with other rare earth minerals like monazite and xenotime. Carbonatite complexes, which are rare igneous rock formations composed mainly of carbonate minerals, are the most significant primary sources of bastnäsite. Examples of such deposits include the Bayan Obo deposit in China and the Mountain Pass deposit in the United States. - Secondary Deposits:
Secondary bastnäsite deposits form through the weathering and alteration of primary deposits. When primary carbonatite or alkaline rocks undergo chemical weathering, bastnäsite can be concentrated in residual soils or alluvial placers. These secondary deposits are typically found in regions where the primary deposits have been exposed to prolonged weathering. In such cases, bastnäsite can be found along with other weathered minerals in lateritic soils or in stream beds where heavy minerals accumulate.
Major Global Locations
Bastnäsite is found in several key locations worldwide, where it is often mined as a primary source of rare earth elements. The major global deposits include:
- China:
China is the world’s largest producer of bastnäsite and other rare earth elements. The most significant deposit is at Bayan Obo in Inner Mongolia, which is the largest known bastnäsite deposit in the world. This deposit is hosted in a giant carbonatite complex and is a major source of light rare earth elements such as cerium, lanthanum, and neodymium. Other notable Chinese deposits include the Weishan and Mianning deposits. - United States:
The Mountain Pass deposit in California is one of the most prominent bastnäsite deposits outside China. Discovered in the 1940s, Mountain Pass was a leading global source of rare earth elements from the 1960s to the early 2000s. The deposit is associated with a carbonatite intrusion and contains high concentrations of light rare earth elements. After a period of closure, mining resumed at Mountain Pass in 2012 to meet the growing demand for rare earth elements. - Canada:
Canada hosts several bastnäsite-bearing deposits, particularly in carbonatite complexes. Notable examples include the Nechalacho deposit in the Northwest Territories and the Strange Lake deposit in Quebec. These deposits are significant sources of both light and heavy rare earth elements, and they are being explored for potential development to diversify the global supply of rare earths. - Madagascar:
The Ambatofinandrahana deposit in Madagascar contains significant concentrations of bastnäsite associated with carbonatite and alkaline igneous rocks. This deposit, although not as large as those in China or the United States, contributes to the global supply of rare earth elements. - Greenland:
Greenland is home to the Kvanefjeld and Tanbreez deposits, which contain bastnäsite and other rare earth-bearing minerals. These deposits are part of the Ilímaussaq alkaline complex and are rich in both light and heavy rare earth elements. Greenland’s deposits are attracting international attention due to their potential as new sources of rare earth elements. - Australia:
In Australia, bastnäsite is found in several locations, including the Mount Weld deposit in Western Australia. Mount Weld is one of the highest-grade rare earth deposits in the world and is being actively mined. The deposit is hosted in a deeply weathered carbonatite and is notable for its high concentrations of both light and heavy rare earth elements.
These locations highlight the geological diversity of bastnäsite occurrences, ranging from primary deposits in carbonatite complexes to secondary concentrations in weathered soils and alluvial deposits. The global distribution of bastnäsite is critical to the supply of rare earth elements, especially in a world increasingly dependent on these materials for high-tech applications and renewable energy technologies.
Applications and Uses of Bastnäsite
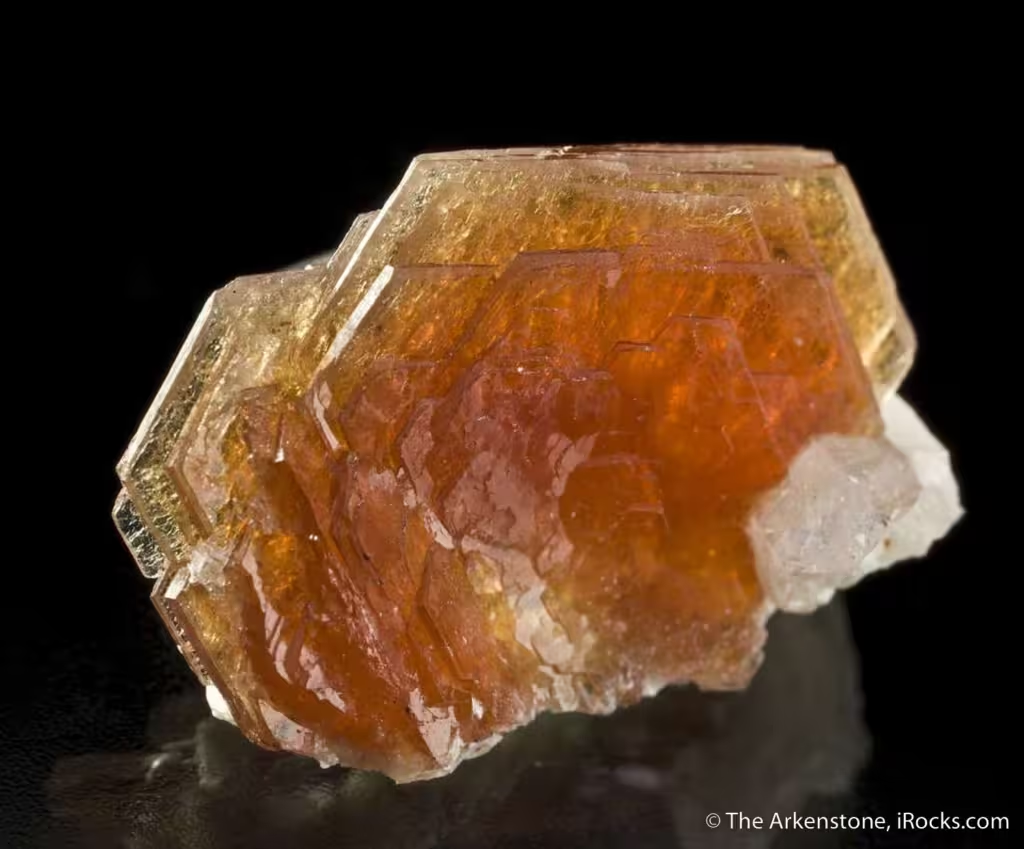
Source of Rare Earth Elements for Various Industries
Bastnäsite is one of the primary sources of light rare earth elements (LREEs) such as cerium, lanthanum, and neodymium, which are crucial for various high-tech and industrial applications. These rare earth elements extracted from bastnäsite are used in several key industries:
- Electronics:
Rare earth elements from bastnäsite, such as neodymium and praseodymium, are essential for the production of high-performance magnets used in electronic devices like smartphones, headphones, and computer hard drives. Cerium and lanthanum are also used in the manufacture of special glass for optical lenses and camera sensors, as well as in polishing powders for refining glass surfaces. - Magnets:
One of the most important uses of rare earth elements from bastnäsite is in the production of neodymium-iron-boron (NdFeB) magnets. These magnets are the strongest type of permanent magnets available and are widely used in various applications, including electric vehicle motors, wind turbine generators, and hard disk drives. Neodymium extracted from bastnäsite is a critical component in these powerful magnets due to its high magnetic strength and resistance to demagnetization. - Glass and Ceramics:
Cerium oxide, obtained from bastnäsite, is used in glass polishing and decolorizing. It serves as a polishing agent for precision optics and glass surfaces. Additionally, cerium and lanthanum are used to improve the optical properties of glass, making it more resistant to ultraviolet light and enhancing its clarity. In ceramics, these elements contribute to the production of specialized glass and ceramic products with high resistance to thermal shock and chemical corrosion.
Role in Renewable Energy Technologies
Bastnäsite plays a vital role in the advancement of renewable energy technologies through its rare earth elements:
- Wind Turbines:
Rare earth elements like neodymium and dysprosium, which can be sourced from bastnäsite, are essential for producing permanent magnets used in the generators of wind turbines. These magnets are crucial for the efficiency and reliability of direct-drive wind turbines, which do not require a gearbox and thus offer reduced maintenance and higher efficiency. This has made rare earth elements indispensable for the growth of wind energy as a sustainable power source. - Electric Vehicles (EVs):
Neodymium from bastnäsite is used in the production of powerful, lightweight magnets essential for electric vehicle (EV) motors. These rare earth magnets provide high torque and efficient performance, which are critical for the operation of EVs. The growing adoption of EVs as part of global efforts to reduce carbon emissions has increased the demand for bastnäsite as a source of rare earth elements. - Solar Panels:
Some rare earth elements obtained from bastnäsite, like lanthanum and cerium, are used in specific coatings and phosphors for solar panels. These materials can enhance the efficiency of photovoltaic cells by improving light absorption and conversion rates, thus contributing to more effective solar energy harvesting.
Use in Catalysts and Phosphors
Bastnäsite-derived rare earth elements are also used in the production of catalysts and phosphors:
- Catalysts:
Cerium, a rare earth element extracted from bastnäsite, is widely used in automotive catalytic converters. These catalysts help reduce harmful emissions from vehicles by converting toxic gases, such as carbon monoxide (CO), hydrocarbons (HC), and nitrogen oxides (NOx), into less harmful substances like carbon dioxide (CO₂) and nitrogen (N₂). Cerium-based catalysts are also used in the petroleum industry for fluid catalytic cracking (FCC), a process that breaks down large hydrocarbon molecules into more valuable gasoline and diesel components. - Phosphors:
Rare earth elements such as europium, terbium, and yttrium, often found in bastnäsite deposits, are key components in phosphors. These materials are used in the production of light-emitting diodes (LEDs), fluorescent lamps, and display screens for televisions, computers, and smartphones. Phosphors made from rare earth elements provide bright, long-lasting, and energy-efficient lighting and displays.
Bastnäsite is a crucial mineral for numerous high-tech and industrial applications. It is a primary source of rare earth elements that are essential for producing advanced electronics, powerful magnets, glass and ceramic products, renewable energy technologies, automotive catalysts, and phosphors for lighting and displays. As global demand for these applications continues to grow, bastnäsite’s importance as a strategic resource in the supply chain of rare earth elements will likely increase, underscoring its critical role in modern technology and sustainable energy solutions.
Processing and Refinement of Bastnäsite
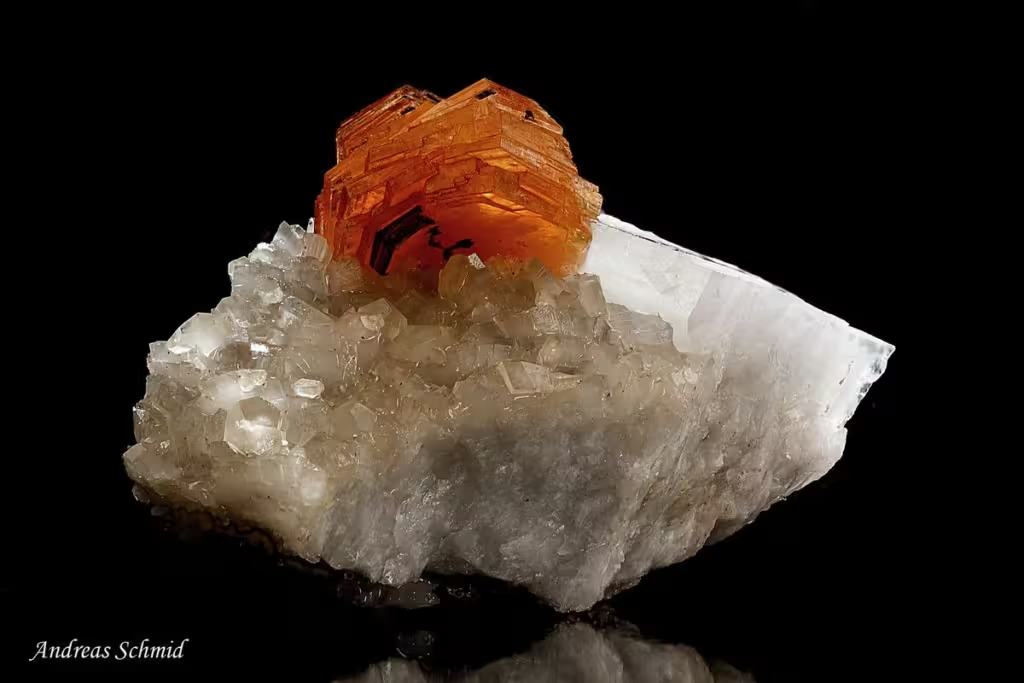
The processing and refinement of bastnäsite to extract rare earth elements (REEs) involve several stages, including mining, beneficiation, chemical extraction, separation, and purification. Due to its complex mineralogy, the processing of bastnäsite requires specialized techniques to efficiently recover the valuable rare earth elements while minimizing environmental impact.
1. Mining and Beneficiation
- Mining:
Bastnäsite is typically mined from open-pit or underground mines, depending on the deposit’s depth and geological characteristics. Once extracted, the raw ore is transported to a processing facility for further treatment. - Beneficiation:
The first step in processing bastnäsite ore is beneficiation, which aims to concentrate the rare earth minerals and remove unwanted materials like silicates and carbonates. This is achieved through a combination of physical methods such as crushing, grinding, and flotation.- Crushing and Grinding: The ore is crushed into small particles to liberate the bastnäsite mineral from the surrounding rock. This is followed by grinding, which reduces the particle size further to enhance the efficiency of the subsequent separation processes.
- Flotation: The ground ore is subjected to froth flotation, where chemicals (collectors, frothers, and modifiers) are added to selectively attach the bastnäsite particles to air bubbles. The bubbles rise to the surface, forming a froth that can be skimmed off, concentrating the bastnäsite while discarding the waste material. This results in a bastnäsite-rich concentrate that typically contains 60-70% rare earth oxides (REOs).
2. Chemical Extraction and Decomposition
- Roasting and Acid Leaching:
The bastnäsite concentrate is often roasted at high temperatures (600-800°C) to remove carbonates, fluorides, and any remaining gangue minerals. This process also converts cerium, a major component of bastnäsite, from its trivalent state (Ce^3+) to its tetravalent state (Ce^4+), which facilitates its removal in subsequent steps. After roasting, the material is subjected to acid leaching (typically with hydrochloric acid or sulfuric acid) to dissolve the rare earth elements into solution while leaving the insoluble impurities behind. - Precipitation and Solvent Extraction:
The leachate, which contains a mixture of rare earth chlorides, undergoes several stages of precipitation to remove unwanted elements like iron, aluminum, and calcium. The purified solution is then treated using solvent extraction, a process where organic solvents selectively bind to specific rare earth elements, separating them based on their different chemical affinities. This process is repeated multiple times to achieve a high level of separation and purity for each individual rare earth element.
3. Separation and Purification
- Ion Exchange:
After solvent extraction, the remaining solution may be further refined using ion exchange techniques. Ion exchange resins selectively adsorb specific rare earth ions from the solution, allowing for the further separation of closely related elements such as neodymium and praseodymium. This method is particularly useful for achieving high purity levels needed for specialized applications, such as in electronics and optics. - Calcination and Reduction:
The separated rare earth compounds are then precipitated, filtered, and dried to produce rare earth oxides. These oxides can be further refined through calcination, a process that involves heating them to high temperatures to remove any residual moisture, organic materials, or other impurities. To produce metallic rare earths, the oxides are subjected to a reduction process, such as electrolysis or metallothermic reduction, typically using calcium or aluminum.
4. Environmental Considerations
Processing bastnäsite to extract rare earth elements involves handling hazardous chemicals and generating significant waste, including acidic wastewater, radioactive residues (if thorium is present), and solid waste materials. To mitigate these impacts:
- Waste Management: Tailings from flotation, residues from leaching, and effluents from solvent extraction processes must be carefully managed to prevent environmental contamination.
- Recycling and Recovery: Developing closed-loop systems to recycle reagents and recover rare earths from waste streams is increasingly important to reduce resource consumption and waste production.
- Radioactive Element Handling: In some bastnäsite deposits, thorium or uranium may be present in small quantities, requiring special measures for safe disposal and containment of radioactive materials.
Conclusion
Processing and refining bastnäsite to extract rare earth elements is a complex, multi-step process that involves physical beneficiation, chemical extraction, and sophisticated separation techniques. While the process is energy and resource-intensive, advancements in extraction and refining technologies continue to improve the efficiency, cost-effectiveness, and environmental footprint of bastnäsite processing, which is vital for maintaining a sustainable supply of rare earth elements essential to modern industries and renewable energy technologies.
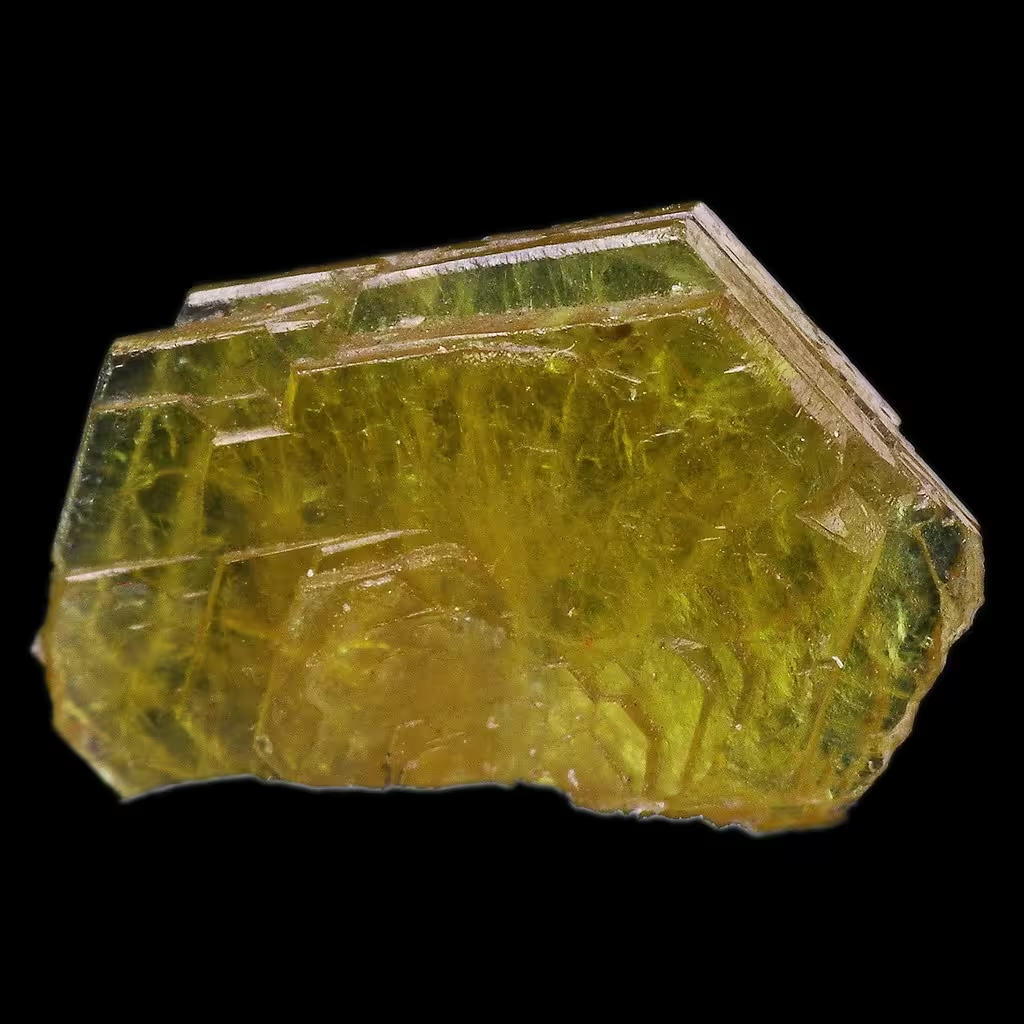